TSUKUBA FUTURE
#067 Fluorescence Bioimaging for Seeing through Living Animals
Assistant Professor MIWA Yoshihiro, Faculty of Medicine
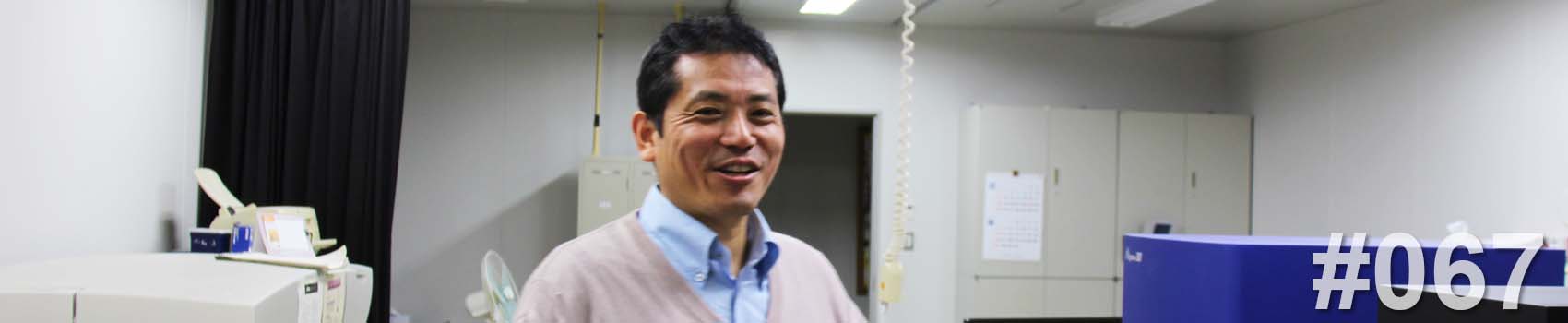
X-ray photography revolutionized medicine. A range of imaging technologies, such as CT scans, ultrasound, and MRI, have subsequently been developed. However, these can only create images of organs and bones. The ability to observe, from outside the body, the functions of individual living cells would surely lead to dramatic developments in medicine. Prof. Miwa is working on the development of this dream technology: fluorescence bioimaging.
The 2008 Nobel Prize in Chemistry was awarded jointly to Osamu Shimomura (Eminent Professor at University of Tsukuba), Martin Chalfie and Roger Y. Tsien for "the discovery and development of the green fluorescent protein, GFP." Prof. Chalfie used the genes for a fluorescent protein which appears green when exposed to ultraviolet light, discovered in the crystal jellyfish by Prof. Shimomura in 1961, to develop a methodology for embedding the protein in living organisms and making them emit light. Prof. Miwa says that he was astounded when he read the paper by Prof. Chalfie published in the journal Science in 1994. Having just graduated from graduate school and found a job at a university, it was this article which helped him map out the future direction of his research.
The conventional method used in research into cell function biochemistry is to grind and examine a clump of cells in a petri dish. However, this method can at most calculate average values as essentially diverse cell groups, in which cells are at different stages of the division process (the cell cycle), are mashed together. Prof. Miwa, frustrated by this difficulty, saw great potential in the use of the fluorescent protein for examining the genetic on/off switches in individual cells. What is more, with this method living cells can be observed. This triggered Prof. Miwa's research into fluorescence imaging.
Prof. Miwa's ambitions subsequently grew even further. He came up with the idea of using fluorescence imaging to research living mice at the individual level, rather than at the cell level. The problem is that visible light (light of wavelengths 380-650 nm [1 nm = 0.000001 mm]) does not reach the outside of the body because it is absorbed by hemoglobin, the pigment in red blood corpuscles, and other substances. As a result, fluorescent substances in the body cannot be seen from the outside even if they are lit up. The fluorescent protein, which radiates green light, is completely invisible. However, 650-900 nm wavelength near-infrared light is not absorbed easily and therefore permeates ten times deeper than visible light. On the other hand, light of even longer wavelengths is absorbed by cell water content. Mice are small so the penetration abilities of near-infrared light are sufficient.
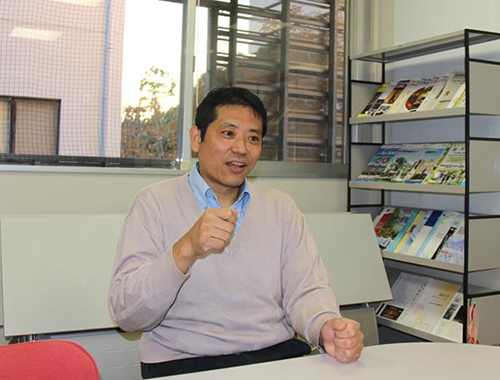
Prof. Miwa always speaks passionately and enthusiastically about his research. He is also proactive in designing outreach programs for primary, junior high and senior high schools to nurture the next generation of scientists.
When observing mice with near-infrared light, the alimentary canal showed up brilliantly because of food the mouse had eaten, however, lesions could not be identified due to only giving off faint fluorescence. A joint venture with food producers was therefore needed to develop food which did not glow under near-infrared light. An examination of all foods on the market at the time found one type which did not glow, but mice given this food appeared emaciated. It took three years to develop a sufficiently nutritious food which did not glow under near-infrared light. Joint research into the development of measurement devices also faced difficulties. Immediately after developing a device specifically designed for mice, the manufacturer decided to pull out of the development of measuring devices for laboratory animals. The laboratory faced the unfortunate setback of having measuring devices confiscated.
Furthermore, in addition to the development of proteins and fluorescent pigment which glow under near-infrared light, it was necessary to prepare a strain of mice which only glow under certain conditions. For example, there was a line of genetically modified mice with a propensity for arteriosclerosis, but it had always been necessary to dissect the mice in order to determine whether or not arteriosclerosis had occurred because the tiny blood vessels were not visible in X-ray images. However, affected blood vessels would be visible using fluorescence imaging, and the progression of the disease could be monitored on the same live individual. At present, Prof. Miwa succeeded in developing mice for three different diseases, in which affected areas glowed only following the onset of the condition, and this is being carried out in tandem with the development of treatment methods. The results of this research will be published soon.
Prof. Miwa has one further ambition in addition to applied research. For example, the liver consists of a number of cell types including liver cells, the cells that make up blood vessels, and others. When it develops, the liver is formed though the interaction of all these different cell types. If each type of cell is marked with a different pigment, it should be possible to track this interaction between the different types of cells. Prof. Miwa calls this the mapping of cell-communities and living systems. Prof. Miwa is determined to develop the tools needed to achieve this and to expand the community of researchers.
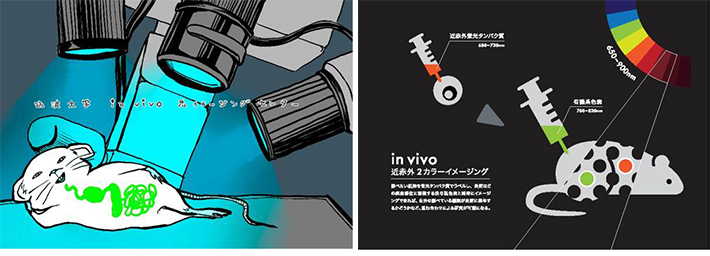
Science illustration lessons are offered in collaboration with Associate Professor Sayoko Tanaka from the School of Art and Design. These pieces explain near-infrared fluorescence imaging. Left: By 1st-year art student Misaki Shibata (2009). Right: By 3rd-year art student Saaya Kuroki (2012).
Article by Science Communicator at the Office of Public Relations